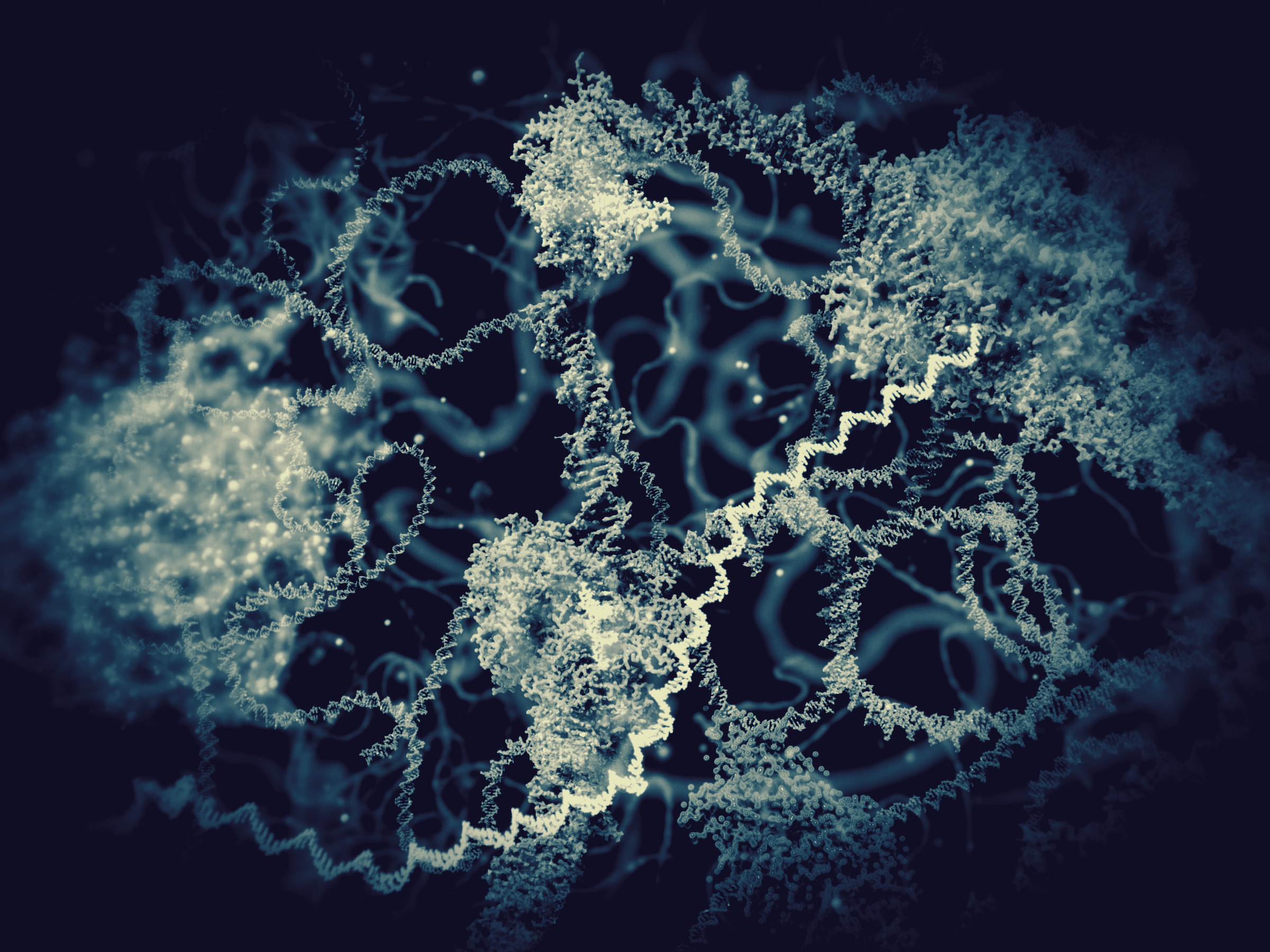
Projects
We study many-body problems in biology at the single-molecule level. Various methodologies developed in the field of single-molecule biophysics allow for studying one molecule’s action in great mechanistic detail, e.g. one RNA polymerase transcribing DNA. However, inside a cell, many proteins work together at the same time, often creating many-body problems. We are curious to know whether proteins show any “collective” behaviors or new characteristics that are not present when a protein works alone. Is the whole greater than the sum of its parts? How? And why?
Traffic phenomenon in the genome
Inside cells, multiple RNA polymerases transcribe a gene at the same time, indicating the presence of RNA polymerase “traffic”, like that of vehicles on the road. When a car stops on a single-lane road, the trailing cars pile up, creating a traffic jam. However, it remains poorly understood what happens when an RNA polymerase pauses while there are multiple RNA polymerases traveling on a DNA template. There are three scenarios, which are not mutually exclusive: (1) hard-sphere interactions between RNA polymerases, similar to a pile-up of cars behind (examined in Kim and Jacobs-Wagner, Biophys J 2018), (2) RNA polymerases pushing the paused one upon collision (Epshtein and Nudler, Science 2003), and (3) dissociation of RNA polymerases (pre-mature termination) upon collision. We aim to accurately characterize the traffic pattern of RNA polymerases and understand the underlying mechanism governing the creation and resolution of the traffic phenomenon.
DNA supercoiling and transcription
Transcription, the first step of gene expression done by RNA polymerase, is inseparable from a mechanical property of DNA, called supercoiling. During transcription, the translocation of an RNA polymerase produces DNA supercoils (twin-supercoiled-domain model by Liu and Wang, PNAS, 1987), and DNA supercoiling, in turn, inhibits the translocation of an RNA polymerase. What if there are multiple RNA polymerases moving on the DNA? Our work in E. coli suggested that neighboring RNA polymerases can affect each other’s speed via the supercoiling state of the inter-connecting DNA, leading to collaborative or antagonistic “group dynamics” of RNA polymerases (Kim, et al. Cell 2019). This finding suggests the ability of DNA to transmit molecular information to a far distance, like a wire transmitting electricity! We investigate the biophysical mechanism of RNA polymerase group dynamics in vitro and in vivo by developing new single-molecule assays. This work will help to begin quantitative and predictive narratives about long-range interactions between RNA polymerases in the genome.
Image credit: Chris Maffeo
The central dogma of molecular biology in the space of a single cell
RNA polymerase transcribes DNA into mRNA, which serves as a template for the ribosome, the protein production machinery. mRNA has a finite lifetime, due to degradation by ribonuclease. The accurate regulation of gene expression is essential for most aspects of cellular life, and the misregulation of gene expression leads to cellular malfunctions and diseases. We study how the functions of RNA polymerase, ribosome, and ribonuclease are orchestrated in space and time within single cells. We hypothesize that the dynamic and transient interactions between these gene expression machines have regulatory outcomes in gene expression. We try to develop single-molecule assays to follow individual molecules’ dynamics in live cells and probe how transcription, translation, and mRNA degradation processes are coordinated in time and space of a single cell.
Emergent properties of DNA loci in live cells
A DNA locus within a chromosome exhibits various dynamic motion that differ widely in time and length scales. We are interested in the spring-like motion of DNA loci happening within a second-to-minute time scale, indicating the polymer property as well as the effect of the surrounding medium. We use the single-particle tracking analysis from soft matter physics and aim to characterize emergent properties of DNA in live cells.
Single molecule imaging at high spatial and temporal resolution
We use single-molecule fluorescence microscopy to study the location, motion, and function of individual molecules in vivo. In particular, we are interested in leveraging advancements in super-resolution microscopy to achieve higher spatial and temporal resolution. Our recent focus is on utilizing the cutting-edge MINFLUX microscope (Abberior) at IGB for both structural and dynamic analyses of biomolecules in bacteria.